Jacobs University, Bremen
Videos
Loading the player ...
- Offer Profile
The Robotics group started at the very
beginning of academic activities at Jacobs University in fall 2001. Much like
Jacobs itself as a novel, highly selective institution in the German university
landscape, robotics is still a very young and very small but ambitious group
comitted to excellence in research. The group is truly international with
English as working language. Jacobs University was called International
University Bremen (IUB) before 2007 [more info]; hence the two names for the
same institution can be found on this website.
Jacobs Robotics is embedded in the EECS undergraduate teaching activities and in
the CS graduate program SmartSystems.
The research of the group focuses on Autonomous Systems. The expertise in this
field ranges from the development of embedded hardware over mechatronics and
sensors to high-level software. On the basic research side of autonomous
systems, machine learning and cooperation are core themes of robotics research
at Jacobs University. The systems developed here are used in various domains,
the most important one being safety, security and rescue robots (SSRR). |
Product Portfolio
Search and Rescue Robots
- Rescue Robots: What is it all about?
Rescue Robotics is a newly emerging field dealing with systems that support
first response units in disaster missions. Especially mobile robots can be
highly valuable tools in urban rescue missions after catastrophes like
earthquakes, bomb- or gas-explosions or daily incidents like fires and road
accidents involving hazardous materials. The robots can be used to inspect
collapsed structures, to assess the situation and to search and locate
victims. There are many engineering and scientific challenges in this
domain. Rescue robots not only have to be designed for the harsh
environmental conditions of disasters, but they also need advanced
capabilities like intelligent behaviors to free them from constant
supervision by operators.
The idea to use robots in rescue missions is supported since several years
by RoboCup, a well-known international research and education initiative in
Artificial Intelligence (AI) and robotics. Already in the summer of 2000,
there was a demo event at the RoboCup world championship in Melbourne,
Australia where the potential of this field was demonstrated. RoboCupRescue
is since then a regular part of RoboCup with dozens of teams applying to
participate in the real robot and simulation competitions. The constantly
increasing high popularity of this field can be explained by the strong
application potential of currently existing systems, which in addition still
leave substantial room for further improvements through basic research on
core issues of AI and robotics.
The Robotics Group at the Jacobs University is since
2001 actively engaged in this research field. The Jacobs Robotics Rescue Robots Team
features several robots, which are complete in-house designs ranging from
the basic mechatronics to the high-level behavior software. Jacobs Robotics also has a special training site, the
Jacobs Robotics Rescue Arena, where the robots and
their operation can be tested in a simulated disaster scenario. 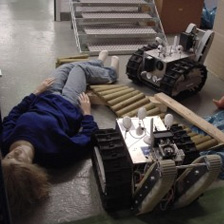
rugbot
- The so-called rugbot type of robot is the latest
development of Jacobs University robotics. The name rugbot is derived from
rugged robot. This robot type is a complete in-house development based on
the so-called CubeSystem, a collection of hardware and software components
for fast robot prototyping. Rugbots are tracked vehicles that are
lightweight (about 35 kg) and have a small footprint (approximately 50 cm x
50 cm). They are very agile and fast on open terrain. An active flipper
mechanism allows Rugbots to negotiate stairs and rubble piles.
Their small footprint is highly beneficial in indoor scenarios. They have
significant on-board computation power and they can be equipped with a large
variety of sensors. The standard payload includes a laser-scanner,
ultrasound sensors, four cameras, one thermo camera, and rate gyros. The
onboard software is capable of mapping, detection of humans and fully
autonomous control. Teleoperation is in varying degrees is also supported.
The on-board batteries allow for 2.5 to 3 hours of continuous operation.
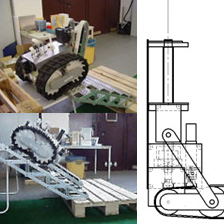
Locomotion
- Rugbots like many other robots designed for operation in
rough environments use tracks. This type of locomotion is often considered
as the most versatile locomotion system as it can handle relatively large
obstacles and loose soil. The technology for tracked locomotion is well
understood and simple. The positive points are smooth locomotion on
relatively smooth terrain, superb traction on loose ground, the ability to
handle large obstacles as well as small holes and ditches, as well as a good
payload capacity. For the negative points, there is slip friction when the
vehicle must turn. Also, if the locomotion has only one pair of belts, it
will suffer from impacts, e.g. when climbing over large boulders 2 or when
it starts going down steep slopes. Nevertheless, tracked locomotion is the
most suitable to surmount obstacles, negotiate stairways, and it is able to
adapt to terrain variations.
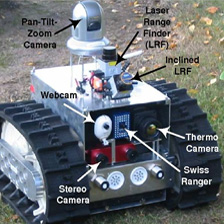
Sensors and Onboard Intelligence
- The rugbots have significant onboard computation power
and various sensor payloads. They can be teleoperated or even run fully
autonomous missions in challenging domains like rescue robotics. The
development of intelligent behaviors is in addition to the robots’
mechatronics a great challenge in this research area.
The robot must for example autonomously detect victims and hazards. Special
sensors like CO2 detectors and Infrared cameras are used to detect humans.
Within the thermo images, a special approach is for example used to detect
human shapes.
The onboard software of rugbots also covers mapping, exploration and
planning. The related research from Jacobs Robotics includes 3D mapping in
unstructured environments.
Last but not least, the onboard software enables rugbots to cooperate in
multi-robot-teams.
Underwater Robotics: The “Lead Zeppelin”
- Underwater robotics is a relatively new domain for us.
Some first successful work includes the development of the Lead Zeppelin, an
autonomous underwater vehicle (AUV).
The “Lead Zeppelin” is an Autonomous
Underwater Vehicle (AUV) developed by the Jacobs Robotics Group and the
Robotics Club in cooperation with ATLAS Elektronik. It is designed for basic
research and education purposes. The “Lead Zeppelin” has already performed
its first major test by participating at the Student Autonomous Underwater
Challenge - Europe (SAUC-E) from the 3rd to the 7th of August, 2006. SAUC-E
was held at Pinewood Studios near London, where a large-scale underwater
stage, which is normally used for movie productions like e.g. James Bond
films, was available for the competition. The Jacobs Robotics team was a real outsider
among the seven participating teams, which all except us had experience with
underwater robotics. Nevertheless, the Jacobs Robotics team came in second in
performance.
The Jacobs Robotics “Lead Zeppelin” is to a large extent a converted land robot as a
major part of its hard and software was developed for the latest generation
of the Jacobs Robotics rescue robots. The most basic underwater parts, namely the hull
with the motors and propellers as well as some of the sensors, were provided
by ATLAS Elektronik. These parts are from a so-called Seafox submarine,
which is a remotely operated vehicle (ROV), i.e., a human steers this
device. The “Lead Zeppelin” got completely new electronics developed at
Jacobs Robotics,
a powerful on-board computer, additional sensors including cameras, and of
course a large amount of software to be able to execute missions on its own.
It is for example programmed to move through an environment and to avoid
obstacles, to recognize targets by computer vision, and to operate at
specific desired depths.
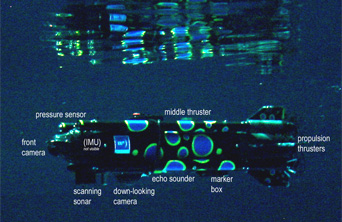
Hardware Components of Lead Zeppelin
- Some of the most basic hardware parts, namely the hull,
the motors and some of the sensors are based on parts from a so-called
Seafox ROV by ATLAS Elektronik. For autonomous operation, completely new
electronics, additional sensors, a high performance on-board computer, and
of course a lot of software was added. The "Lead Zeppelin" AUV uses two
computation units, which is a common approach for so-called CubeSystem
applications. The basic hardware control is done by a RoboCube. Higher level
AI software is running on an embedded PC. It collects all sensor data from
the RoboCube as well as from the sensors directly attached to it. On an
external operator PC, which is connected via wireless network, a GUI is used
that can be used for teleoperation and to debug autonomy. There is the
option to use an antenna-buoy to maintain wireless communication. This is of
course not needed if full autonomy is activated.
The submarine is composed of a main hull, a nose and four long tubes. Those
parts house the power system, the propulsion system, a low level controller,
a high level controller and a rich sensor payload.
The nose section contains:pressure sensor for depth measurement
heading sensor including pitch and roll sensor
scanning sonar head
front camera
The middle section contains:
- echo sounder
- vertical thruster
- embedded PC
- RoboCube
- DC/DC converters
- wireless access point
Outside, below the middle section the following parts are attached to the
vehicle:
- down-looking ground camera
- marker disposal system
Furthermore, four battery tubes are attached to the middle section, each
containing:
- propulsion motor
- propeller with guards
- power amplifier for the motor battery
The submarine is trimmed slightly positively buoyant, i.e. it comes up to
the surface in case of some error. Thus the middle thruster has to be used
to force the vehicle under water. The maximum power of the four propulsion
motors is 350 W of which up to 280 W are usable due to PWM duty cycle
limitations. The 3-blade propellers are protected by guards for safety.
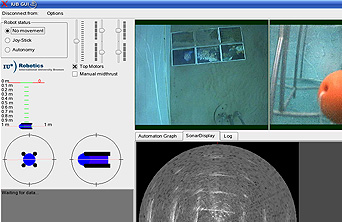
Title
- A. Heading Sensor
A MTi IMU from Xsens is used. It is a low-cost miniature inertial
measurement unit with an integrated 3D compass. It has an embedded processor
capable of calculating the roll, pitch and yaw in real time, as well as
outputting calibrated 3D linear acceleration, rate of turn (gyro) and
(earth) magnetic field data. The MTi uses a right handed Cartesian
co-ordinate system which is body-fixed to the device and defined as the
sensor co-ordinate system. Since the gyro is placed near the center of the
sub the orientations provided by the device can directly be used as vehicle
orientations. The heading sensor is used to measure and control the roll,
pitch and yaw of the AUV.
B. Scanning Sonar
The submarine has a scanning sonar which enables it to scan the surrounding
for obstacles and their distance by emitting sound signals of 550 kHz and
measuring the time it takes until the sounds echo comes back. The sonar
covers up to 360 degrees since it is being turned by a motor in steps of one
degree or bigger and it has a resolution of 1.5 deg. The range of the sonar
is selectable to up to 80 meters. The sensor can also scan vertically with a
coverage of ± 20deg. The horizontal scan rate is about 60deg per second at
distances of up to 10 m.
C. Pressure sensor
The pressure sensor measures the depth of the submarine below the water
surface. It has an operating pressure range from 0 to 31 bar thus being
operational to depth of 300 meters. It can be directly interfaced to the A/D
converters of the RoboCube.
D. Echo sounder
The echo sounder measures the distance from the submarine to the ground by
emitting sound pulses with a frequency of 500 kHz and a width of 100 µs. The
echo of this sound signal is being received and the travel time measured is
then used to calculate the depth. The beam width is ± 3deg, the accuracy ± 5
cm and the depth range 0.5 to 9.8 meters.
E. USB cameras
Two Creative NX Ultra USB cameras are used in the vehicle. They support a
resolution of up to 640 x 480 pixel. Those USB 1.1 devices have a wide-angle
lens which enables a field of view of 78deg. The camera at the bottom of the
submarine looks at the ground. It can for example be used to locate targets
and to guide the submarine directly over them. The front camera can be used
to search for the bottom as well as the mid-water targets. Both cameras are
inserted in a waterproof protective lid of transparent plastic.

The AUV Software
- The software for the "Lead Zeppelin" AUV is structured
like in other CubeSystem robots in two parts. The basic low level control is
done on the RoboCube. It uses the CubeOS and the RobLib to generate the
proper PWM signals, to decode the encoder signals from the motors and to
access the analog pressure and echo sound sensors. The higher level AI
software is running on the robot PC, which uses SuSE 10 as operating system.
It collects all sensor data from the cube, especially depth and speed, as
well as from the sensors directly attached to the PC’s interfaces. It uses
the high-resolution sonar head to do obstacle avoidance
and localize other objects in the basin. The front USB camera can be used to
find for example midwater targets. The down-looking camera can for example
locate targets situated on the bottom. The software reuses quite some
functions and libraries developed for other Jacobs Robotics robotics projects,
especially the rescue robots.
The AI software is embedded in a robot-server, which is a multi-threaded
program written in C++. All system-wide constants like port numbers,
resolutions, etc., are read at startup from a configuration file. A client
GUI running on a PC or a Laptop is connecting to this server in order to
manually drive the robot to a start position using a Gamepad, to start the
autonomy and to observe the submarines status during the mission if wireless
connection is still available. The NIST RCS framework is used to handle
communication between the robot-server and the operator GUI. This framework
allows data to be transferred between processes
running on the same or different machines using Neutral Message Language (NML)
memory buffers. All buffers are located on the robot computer, and can be
accessed by the operator GUI process asynchronously. The GUI uses Trolltechs
cross-platform Qt Class Libraries. Several threads are running in parallel
to handle the massive amount of data. Each camera view has its own thread.
3D Mapping in Unstructured Environments
- This ongoing project is funded by the Deutsche
Forschung Gemeinschaft (DFG). It deals with the generation of 3D maps with a
mobile robot in unstructured environments like search and rescue scenarios.
The project uses a special learning approach. The goal is to a metric 3D
environment representation encoded in a compact surface model language like
X3D, the successor of the Virtual Reality Modeling Language (VRML). The
algorithm uses a special online evolution where a local X3D neighborhood
model is extracted raw 3D sensor data while the robot moves along. Inspired
by previous successful work on realtime learning of 3D eye-hand coordination
with a robot-arm, the evolutionary algorithm tries to generate compact model
code that reproduce the vast amounts of sensor data.
In doing so, a special metric to compare the similarity of the sensor input
with a candidate model is used. Unlike other approaches using Hausdorff
distance or detecting correspondences between invariant features and using
transformations metrics on them, this similarity function has several
significant advantages. First of all, it can be computed very efficiently.
Second, it is not restricted to particular objects like polygons. Third, it
operates on the level of raw data points. It hence does not need any
preprocessing stages for feature extraction, which cost additional
computation time and which are extremely vulnerable to noise and occlusions.
Last but not least, the project tackles the localization of the robot.
Instead of using standard SLAM approaches, the problem is dealt with by
using map fusion. A second evolutionary algorithm generates different poses
of the local VRML model with respect to the global one and measures the
similarity of overlapping regions. The same special similarity metric used
for the generation of the local model can also solve this task. Based on the
estimations of the pose of the
robot within the global model, the initial population is seeded with
according poses of the local model. In case the robot is severely lost,
there is still a realistic chance that a proper integration is done due to
sufficient similarity of parts of the local model with the right parts of
the global model and the robot gets again correctly localized. 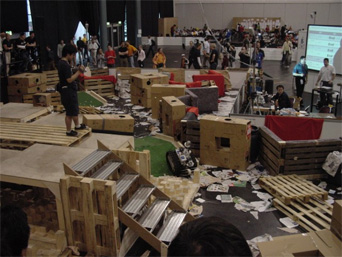
A typical unstructured environment: the Rescue League
arena at RoboCup 2006 in Bremen.
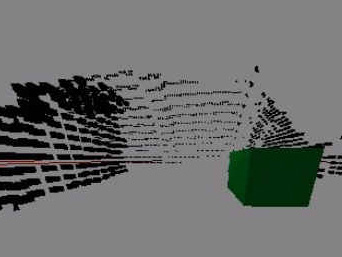
3D model from a stationary robot
3D Wordmodeling for Robot Action Planning, Recognition
and Imitation
- The project deals with the investigation of 3D world
modeling and robot action planning, recognition and imitation. It is based
on joined research at the Jacobs University Robotics group and the
Biologically Inspired Autonomous Robots Team (BioART) lead by Yiannis
Demiris at Imperial College. An other current project at Jacobs Robotics
focuses on the problem of autonomously creating 3D representation of an
unstructured indoor environment by a mobile robot (3D map). The architecture
that is being developed at Jacobs University can extend the system designed
by BioART for robot action perception and imitation by alleviating the
constraints it imposes on the environment. At the same time, the 3D world
modeling framework can be improved significantly by coupling it with an
attentive mechanism, which is part of the architecture developed at BioART.
Such exchange of knowledge will be a significant benefit for both research
groups, resulting in superior performance of their currently developed
systems.
Multi-Robot-Teams: Cooperative Mapping and Exploration
- Multi-robot-teams are beneficial for many application
domains as a group of robots can do many tasks much more robustly and much
more efficiently than a single robot. A particular good example is the field
of Safety, Security and Rescue Robotics (SSRR) as demonstrated with the
Jacobs rescue robots. Especially reconnaissance missions, e.g. the search
for victims in a rescue scenario, can profit from several robots operating
in parallel. But achieving proper cooperation is non-trivial as illustrated
by the following examples of contributions by Jacobs Robotics.
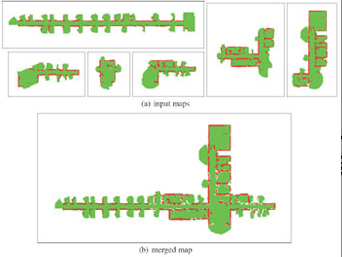
Map Merging
- Mapping can potentially be speeded up in a significant
way by using multiple robots exploring different parts of the environment.
But the core question of multi-robot mapping is how to integrate the data of
the different robots into a single global map. A significant amount of
research exists in the area of multirobot-mapping that deals with techniques
to estimate the relative robots poses at the start or during the mapping
process. With map merging the robots in contrast individually build local
maps without any knowledge about their relative positions. The goal is then
to identify regions of overlap at which the local maps can be joined
together. A concrete approach to this idea was developed by Jacobs Robotics
in form of a special similarity metric and a stochastic search algorithm.
Given two maps m and m', the search algorithm transforms m' by rotations and
translations to find a maximum overlap between m and m'. In doing so, the
heuristic similarity metric guides the search algorithm toward optimal
solutions.
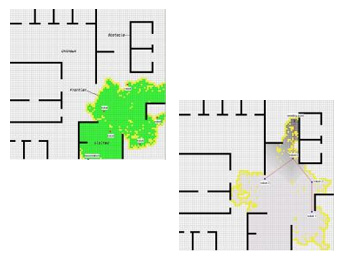
Cooperative Exploration
- A popular basis for robot exploration is the
frontier-based exploration algorithm introduced by Yamauchi in 1997. In this
algorithm, the frontier is defined as the collection of regions on the
boundary between open and unexplored space. A robot moves to the nearest
frontier, which is the nearest unknown area. By moving to the frontier, the
robot explores new parts of the environment. This new explored region is
added to the map that is created during the exploration. Obviously,
multi-robot systems are a very interesting option for exploration as they
can lead to a significant speed-up and increased robustness. There are
several possibilities to extent the frontier based algorithm to a multi
robot version. First of all, there is the option to use the most
straightforward extension; namely to let the robots run individually and to
simply fuse their data in a global map. Each robot then simply moves to its
nearest frontier cell.
More sophisticated versions try to coordinate the different robots such that
they do not tend to move toward the same frontier cell. One can for example
use a utility that incorporates the cost of moving to the frontiers and that
discounts situations where two or more robots approach the same frontier
region. An other often neglected problem for multi-robot exploration is that
the robots need to exchange data. But when it comes to real multi-robot
systems, communication relies on wireless networks typically based on the
IEEE 802.11 family of standards, which is also known as WLAN technology, or
some alternative RF technology. RF links suffer from various limitations.
Especially, they have a limited range. At least, not every robot has to be
within the range of each others transceiver. When using ad-hoc networking,
the underlying dynamic routing can be employed to transport data via several
hops of robots that are in mutual contact to bridge longer distances.
Jacobs Robotics has developed an extension of frontier based exploration,
which takes constraints on the exploration like limited range of radio links
into account. This algorithm is based on a utility function, which weights
the benefits of exploring unknown territory versus the goal of not violating
the constraints like keeping communication intact.
Virtual Robots for Research and Training Applications
- The Jacobs virtual robots are based on USARSim, a
high-fidelity simulation of large scale urban and indoor environments where
different robot platforms can operate in various scenarios. USARsim is an
extension of the Unreal Tournament game engine. The underlying Karma physics
engine provides kinematically correct robot motions, sensors from a rich
selection of standard models can be added to the robots to gather data, and
the different USARsim scenarios cover various environment conditions ranging
for example from difficult terrain as locomotion challenge to smoke from
fires as visual obstruction. The simulator is mainly laid out for Safety,
Security, and Rescue Robotics (SSRR). But it is also well suited for work on
mobile robots at large. It is an ideal tool for testing prototype
implementations of robot algorithms in general. But its main strength from a
research perspective is the possibility to investigate multi-robot teams.
From an application viewpoint, USARsim is a very beneficial tool for
training and exercising.
The virtual robots supplement the real Jacobs rescue robots. Each virtual
robot runs the autonomous software developed by Jacobs Robotics, i.e.,
intelligent functions for perception, control, mapping, planning,
cooperation, and so on.
CubeSystem
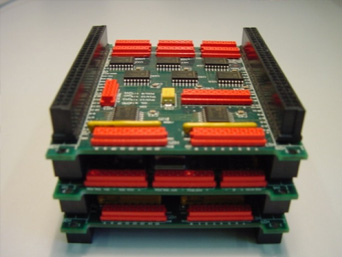
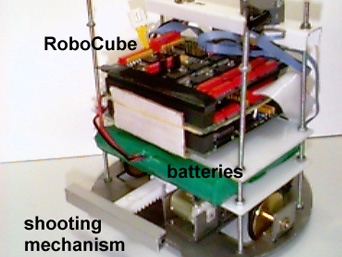
- The CubeSystem is a collection of hardware- and
software-components for fast robot prototyping. The main goal of the
CubeSystem project is to provide an open source collection of generic
building blocks that can be freely combined into an application. The
CubeSystem evolved in more than five years of research and development. The
benefits of the CubeSystem can be seen by various applications in which it
is used. These applications range from educational activities to industrial
projects.
There are various other projects that deal with the development of generic
hardware or software libraries for robotics. These projects are typically
based on the assumption that a robot's hard- and software are two rather
distinct parts, that can be easily brought together by the usage of the
right type of abstractions and interfaces. This view is to quite some extent
valid when it comes to the development of application software for
commercial of the shelf robot hardware. But the development of a robotics
application often includes the engineering of the hardware side as well. The
CubeSystem therefore tries to offer a component collection for fast
prototyping of complete robots, i.e., the hardware and the software side.
The most basic parts of the CubeSystem are:- the RoboCube: a special embedded controller, or more precisely
controller family, based on the MC68332 processor
- the CubeOS: an operating system, or more precisely an OS family
- the RobLib: a library with common functions for robotics